Abstract
At the elementary, secondary, and post-secondary stages of education, the ideas of heat and temperature are crucial to science education. This study set out to look into the difficulties that students have when learning about heat and temperature. A survey containing open-ended, multiple-choice, and Likert-scale questions was utilized by the researchers to gauge the comprehension of 205 participants who the City College of New York students were. The investigation’s findings showed that students had trouble developing a conceptual understanding of the topics of heat and temperature and frequently rely on their own knowledge, reason, and common sense rather than establishing a thorough scientific understanding. Their capacity to apply these concepts in various circumstances is hampered by this conceptual knowledge deficit. The study also discovered that as individuals advance through their academic careers, they typically get a better comprehension of heat and temperature concepts. The results of this study suggest that educators should be aware of these difficulties and work on addressing the need of students to develop meaningful, deep learning of these concepts.
License
This is an open access article distributed under the Creative Commons Attribution License which permits unrestricted use, distribution, and reproduction in any medium, provided the original work is properly cited.
Article Type: Research Article
INTERDISCIP J ENV SCI ED, Volume 21, Issue 2, 2025, Article No: e2510
https://doi.org/10.29333/ijese/15997
Publication date: 01 Apr 2025
Online publication date: 18 Feb 2025
Article Views: 1039
Article Downloads: 552
Open Access HTML Content Download XML References How to cite this articleHTML Content
INTRODUCTION
At its foundation, science is a search to understand the mysteries of the world we live in and the complex processes that underline natural phenomena (McCain, 2015). Instilling in our students a spirit of inquiry and discovery is important to us as educators because it encourages them to look into the fundamental causes of scientific events and come up with their own explanations (Braaten & Windschitl, 2011). The problems we confront in the quickly changing world of today call for creative solutions that are based on scientific inquiry and thought (Hernandez & Edinson, 2021). As a result, a high-quality education must encourage students’ active learning and provide them with the methods and skills necessary to interact with scientific ideas in real-world contexts. Pedagogical content knowledge, which includes both a thorough understanding of the subject matter and the capacity to use effective teaching tactics, representations, and demonstrations to aid student learning, is a crucial component of an effective science education (Shulman, 1986). By giving our students these fundamental abilities, we enable them to become independent thinkers who can create their own knowledge and interact meaningfully and in a transformational fashion with scientific ideas. The students of today, who are aspirant scientists, are the scientists of future, so it is critical that we give them the training and tools they need to be authorities in their field (Mason & Zaccoletti, 2021). We can equip undergraduate science students with the knowledge and confidence to begin their scientific journeys and to embark on a road of lifelong learning and discovery by encouraging an environment of active learning and providing them with a solid foundation in pedagogical topic knowledge.
As Ausubel (1968) famously observed, “The most important single factor influencing learning is what the learner already knows”, the significance of prior knowledge in learning cannot be over emphasized. This is particularly true in the teaching of science, as students frequently carry preconceptions and misconceptions from earlier educational experiences. Even if students can successfully complete rote exercises or algorithmic problems, research in science education has revealed that students may still have difficulty due to weak conceptual underpinnings (Lythcott, 1990). For instance, Peterson and Treagust (1989) discovered that despite performing well on exam questions gauging their understanding of the subject, a sizable portion of secondary school students were unable to react correctly to conceptual questions relating to specific content. These results show that educators must not only impart new knowledge but also recognize and correct students’ misconceptions. In order to create meaningful and long-lasting learning experiences, educators can build on students’ prior knowledge and understanding. In order to assist students to build a strong conceptual framework that supports their learning and comprehension of scientific concepts, it is essential to identify and resolve their misconceptions. One of the most important aspects of science education is how prior knowledge affects learning. To encourage a deeper comprehension of scientific ideas and to create a more productive and interesting learning environment for students, educators should be aware of and responsive to student misconceptions.
The availability of competing concepts can make it difficult for students to develop a conceptual knowledge of science. Students are taught concepts like acids, elements, oxidizing agents, covalent bonds, and chemical shift at various levels, but frequently they arrive with prior knowledge that necessitates considerable conceptual revisions (Chua & Maloles, 2020). Alternative conceptions, misconceptions, or alternative frames are terms frequently used to describe student beliefs that are at odds with generally recognized scientific knowledge (Taber, 2019). In order to effectively apply their knowledge in the future, students must accurately explain, comprehend, and apply scientific concepts (Kramarová & Proksa, 2020). The three basic states of matter–solid, liquid, and gas–are thought to change from one to the other, the addition of oxygen to a reaction results in oxidation, and endothermic reactions cannot be spontaneous, to name a few common misconceptions held by students (Garnett & Treagust, 1992; Schmidt, 1997; Thomas & Schwenz, 1998). These misunderstandings could be the result of flawed thinking or poor intuition. Effective tactics like re-explaining and cognitive conflict are necessary to overcome misconceptions (Permata et al., 2019). Teachers can aid students in gaining a more accurate and thorough comprehension of scientific issues by pointing out and dispelling alternate conceptions. In order to facilitate meaningful learning experiences in science education, it is crucial to encourage a deeper comprehension of topics and offer opportunities for students to reconcile their preconceptions with received scientific information.
People build mental models out of their experiences to help them make sense of the world. They employ these mental models to evaluate the information, draw conclusions, or make predictions in the presence of an uncommon event or phenomenon. Students today need to contribute actively to the teaching and learning process. They need to engage in a variety of exercises to improve their critical thinking abilities. To increase retention, instructors should encourage students to complete their assignments on their own (Chua & Maloles, 2020). Due to their abstract concepts (students find it difficult to believe in things they cannot see), common (non-scientific) word usage, familiarity with daily life (everyday analogies), visible effects and changes, and nuanced concepts, temperature and heat are two topics that students have misconceptions about (Colburn, 2009). Students purposefully avoided using scientific terminology (often known as “fancy scientific words”) in favor of everyday speech, which resulted in several misunderstandings. Teachers should assist students in a critical analysis of the limitations of metaphors and analogies, according to Hesse and Anderson (1992).
Alternative ideas are quite tenacious in chemistry, just like they are in physics. According to Gabel (1999), there are three ways to express matter: macro, sub-micro (in the form of particle models), and symbolically (in the form of chemical notation). Chemistry training frequently emphasizes the abstract and symbolic levels, which can be unproductive. Additionally, as mentioned by Griffiths and Preston (1992) and Novick and Nussbaum (1978), students have a hard time visualizing “nothing” between atoms or molecules. According to studies, children’s alternative conceptions of chemistry evolve quickly between the ages of 6 and 12, but beyond that, they change more slowly and continue until adulthood. For instance, Ahtee and Varjola (1998) discovered that 10% of Finnish eighth graders, along with secondary school and university students, were unable to differentiate compounds from atoms. Similar findings were made by Lewis and Linn (1994), who found that only PhD holders had lower rates of misconceptions regarding thermodynamic concepts than college students and “experts” with advanced degrees in several areas. According to Schmidt (1997), popular word meanings play a key role in producing misunderstandings by helping students form strong mental frameworks that are difficult to modify.
Students frequently make mistakes in learning for simple memorization, which can limit their understanding. Deep knowledge is known to be hampered by an excessive dependence on memorizing (Schullery, 1979). In fact, well-liked learning aids for chemistry, like preprogrammed study guides, in-depth problem manuals, computer-generated homework assignments, and repeatable exams, may unintentionally cater to concrete-operational learners by letting them get by with the material without really understanding the underlying ideas. Instead, the focus should be on encouraging formal-operational thinking so that students are able to independently explain topics without the use of these tools. When Boujaoude (1992) created a tool to classify high school students as “rote learners” or “meaningful learners,” he discovered that both groups initially displayed comparable levels of misunderstanding. The concept mastery of meaningful learners, however, significantly improved following training, whereas rote learners either made no progress or even regressed. Rote learning helps keep different ideas about chemistry alive, along with other elements like reliance on algorithms and static textbooks.
Eric Mazur, a Harvard professor, tested 250 of his students’ knowledge of Newtonian mechanics through an experiment, and the findings shocked him. The students’ inability to respond to simple questions and their memorization of equations and problem-solving techniques revealed a serious lack of understanding of the subject matter. He found that his lectures had no impact. He reconsidered how he lectured [regurgitated the material] and started using more peer assessment. Peer assessment is when two or more students collaborate to accomplish assignments that require nearly equal participation from each participant for the process to be successful. According to Hanrahan and Isaacs (2001), some students feel that taking part in peer evaluation exercises is advantageous because it gives them a better understanding of how teachers assess their students. Peer assessment also has benefits for students in terms of better learning and higher responsibility for others (Papinczak et al., 2007). Peer assessment in the field of education, however, is now in a poor state, resembling a dense forest of isolated case studies and modest, transient experiments. Peer evaluation won’t be able to escape from the shadows and assume its due position as a ground-breaking tool in pedagogy–a beacon of evidence-based practice that has long been desired (Ashenafi, 2017).
Teachers may be frustrated when they discover that students may still learn by memorizing facts and solutions to particular types of problems after realizing the significance of students’ alternative conceptions in a subject area. What should educators do as a result? First and foremost, the instructor should create a curriculum that emphasizes success and is built around the core ideas of the discipline, like chemistry, that students must fully comprehend by the end of the course (Horton, 2007). Less material may be covered as a result, but as long as the students have a solid grasp of the fundamental ideas, this is not harmful. Second, as recommended by Lewis and Linn (1994), common knowledge should be included in the curriculum to foster knowledge integration, inspire students to build on their innate understandings, and simplify scientific material for improved retention. It is crucial to remember that while educators can take these steps to enhance their instruction, it is ultimately the responsibility of the students to learn. Like watering their own garden, they must be responsible for their own education.
Scientific literacy has become an essential qualification for everyone in a world where scientific research affects all parts of our life. A strong understanding of science and scientific processes is crucial in developing these talents, which are increasingly demanded by employers due to the need for advanced learning, reasoning, creative thinking, decision-making, and problem-solving abilities. From elementary to postsecondary education, science courses frequently include ideas about heat and temperature (National Research Council, Board on Science Education, National Committee on Science Education Standards, & Assessment, 1995). Students are able to function effectively because they already have a plethora of knowledge about the world and have built their own framework for comprehending the physical world. True scientific literacy, however, goes beyond simply amazing, isolated facts, as such knowledge is quickly lost when it is no longer regarded as being of any benefit (Harris, 1997). Therefore, acquiring true scientific literacy requires the collection of ideas rather than just information. Finding students’ learning barriers and figuring out the best ways to address them has been a big difficulty in the field of chemistry education research.
The inability of students to comprehend how chemical entities develop, as well as their properties and interactions, is one of the main causes of learning difficulties and misconceptions in chemistry, according to research (Tümay, 2016). However, it has been demonstrated that the employment of cutting-edge methods enhances students’ attitudes and motivation toward this subject. For instance, Zamora-Polo et al. (2019) combined the flipped classroom strategy, in which study materials are accessed outside of the actual classroom, with gamification, which involves using game elements and design techniques in non-game contexts. According to their findings, this educational innovation enhanced student motivation, helped students recognize good teaching techniques, and made students feel better about science education and scientific challenges. In addition, students develop fresh talents that they can use in their upcoming professional activities. Given their potential to have a positive impact on future generations, innovations introduced in a university setting may have a multiplier effect (Zamora-Polo et al., 2019).
Much research in the area of scientific education have focused on investigating students’ misunderstandings or misconceptions of the basic ideas of heat and temperature. Given this, it is essential to use acceptable approaches that clearly communicate the correct scientific ideology associated with these notions (Farooq et al., 2021). For instance, Alwan (2011) conducted a thorough analysis to identify common misconceptions about heat and temperature held by undergraduate science students. The results showed that many students had different beliefs about these notions, which caused confusion and misunderstandings. There were several false beliefs that were discovered, including the incorrect notion that a substance’s boiling point represents the highest temperature it can reach, the false notion that a cold body has no heat, the false notion that an object’s temperature depends on its size, the false notion that heating always raises the temperature, and the false notion that thermal equilibrium is an invalid concept. A lot of students also believed incorrectly that the amount of heat is purely reliant on the object’s temperature, thinking that objects with greater temperatures automatically contain more heat energy (Alwan, 2011).
Similar to this, Thomaz et al. (1995) showed that a sizable portion of secondary students in the UK had trouble understanding the idea of objects maintaining the same temperature when in contact with their surroundings for a lengthy period of time. According to Hewitt (2006), on the other hand, heat moves from things with higher temperatures to those with lower temperatures when they are in contact and have temperature differences. Temperature is a measurement of the average kinetic energy of molecules or atoms. According to Kesidou et al. (1995), heat is primarily linked to energy transfer brought on by temperature differences and is regarded as a process energy form as opposed to a storage energy form in the system. In order to build precise conceptions that may describe commonplace events connected to thermal notions, it is necessary for students to gain a strong scientific grasp of the terms temperature and heat (Chu et al., 2012).
Jasien and Oberem (2002) tested whether there was a correlation between the quantity of college-level physical science courses taken and the proficiency in answering straightforward questions on thermal equilibrium. According to Jasien and Oberem’s (2002) findings, there is no link and there is misunderstanding regarding a variety of concepts, most notably the definition of thermal equilibrium. This study examined how well undergraduate students understood fundamental ideas about heat and temperature, particularly how thermal equilibrium functions. Since these concepts are necessary for comprehending the more difficult thermodynamic concepts, they can be used as a gauge for the framework that students will bring to the study of more sophisticated issues in thermodynamics. The researcher will use a practical approach and give questionnaires to undergraduate students to gauge their conceptual comprehension of concepts linked to heat and temperature.
With this project, we hope to learn more about the difficulties undergraduate students are now facing as well as how confident they feel in responding to the survey’s three multiple-choice questions. By obtaining information from students, this study aims to shed insight on how these fundamental scientific ideas are perceived and understood. This knowledge will be useful for future research and potential interventions to improve students’ learning experiences.
Guiding Research Questions
-
What are some of the learning difficulties that students experience in learning about heat and temperature concepts reactions?
-
What approaches do students use when solving problems related to heat and temperature?
-
How does scholastic achievement correlate with learning about heat and temperature?
METHODS
This research aims to examine the challenges that students face when learning about issues linked to heat and temperature, as well as the methods they use to deal with these concerns. The City College of New York, a public university that provides services to minority communities in an urban environment, was the site of the study. A survey was carried out that included three general chemistry multiple-choice questions, two short answer questions, and four Likert-type questions in order to evaluate the difficulties that students encounter when solving heat and temperature problems. 205 people who represented a wide range of majors, including science, engineering, liberal arts, and undeclared, took the anonymous poll. The administration of the survey and data gathering were approved by the City College of New York Internal Review Board. The survey findings were examined using a variety of visualization techniques, such as graphs to show students’ responses, histograms to display the percentage of correct answers for each multiple-choice question, and line charts to represent the confidence levels of each multiple-choice question.
The responses to the Likert-style questions were transformed into numerical values and plotted, as follows: strongly disagree is followed by disagree, neutral, agree, and finally strongly agree. The responses from respondents were compiled for some of the open-ended questions. The multiple-choice answers from the three general chemistry questions were transformed into percentages and presented as histograms and line graphs. Each multiple-choice question’s confidence level was also converted to a numerical value and plotted, as follows: very high = 1, high = 2, moderate = 3, low = 4, and very low = 5.
Two specialists who reviewed the survey concluded that the questions accurately reflect the inquiry into note-taking and its relationship to learning, performance, and memory. The reliability coefficient was calculated using the test-retest approach and was found to be 0.85. The Likert-type questions were subjected to a single factor ANOVA, which revealed p < .001 and p < 0.05, which is strong evidence against the null hypothesis and demonstrates a strong association between the variables.
According to the Dunning-Kruger (DK) effect, individuals with low ability tend to exaggerate their aptitude. This hypothetical cognitive bias was first discussed in Kruger and Dunning (1999), and if it is real, it has the potential to be both significant and harmful because it would mean that individuals with low abilities not only execute tasks poorly, but also–worse yet–believe that they do so. Kruger and Dunning (1999) mentioned that people with low ability have trouble assessing and seeing themselves (a lack of metacognitive skills). People with high ability tend to underestimate their capacity, which is a closely linked effect that is also significant but perhaps less harmful. Although it is not mentioned in Kruger and Dunning (1999), this second impact is frequently linked to their names. Kruger and Dunning’s (1999) theory of the DK effect has been extensively debated and contested (Magnus & Peresetsky, 2022).
RESULTS AND DISCUSSION
Figure 1 displays the averages of the Likert-type questions. The graph demonstrates that the majority of students frankly admit they don’t have a solid understanding of heat and temperature. Research in scientific education shows there was misunderstanding regarding a number of concepts, most notably the definition of thermal equilibrium (Jasien & Oberem, 2002), which lends support to this. The students honestly state that problems involving heat and temperature are simple to solve and that the professor or teacher well taught heat and temperature principles. On the other hand, students clearly state that in order to solve associated problems, a solid understanding of heat and temperature is necessary. This is in line with studies on science education, which shows that it is essential for students to have a strong scientific knowledge of the terms “temperature” and “heat” in order to create concepts to explain common events that involve thermal notions (Chu et al., 2012).
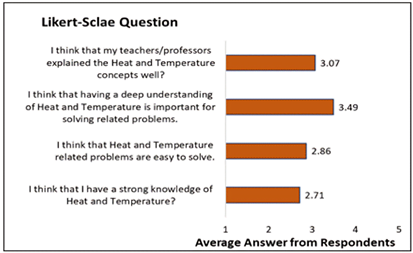
Students were asked open-ended questions about the challenges they had when learning about ideas linked to heat and temperature. These questions were examined and grouped into six major categories. The replies were distributed differently, but the issues with translating between temperature units and a lack of understanding of the concepts of heat and temperature were most frequently mentioned. Figure 2 demonstrates the challenges students have while trying to understand ideas linked to heat and temperature. According to the results, 21.9% of students had trouble understanding the ideas of heat and temperature, and 20.9% had trouble understanding equations that related these ideas. This result is in line with earlier studies by Kesidou et al. (1995), who found that students had difficulty differentiating between heat and temperature in the extensive and intensive frameworks.
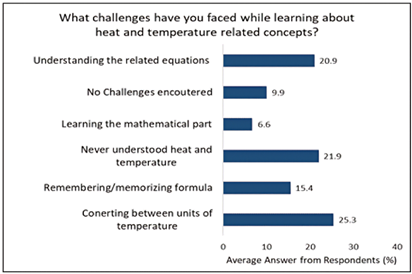
The most difficult part, according to Figure 2, was the conversion between temperature units, which was problematic for 25.3% of students. 15.4% of students had difficulty remembering formulas for resolving heat and temperature-related issues. According to Braaten and Windschitl (2011), it is stressed in science education that students must look into the causes of a phenomenon and come up with their own interpretations. One explanation for this is that chemistry textbooks and other science textbooks often dilute their subject matter to the point that it has no real significance in order to make science attractive, applicable, and intelligible to students (Bucat et al., 1988). The next generation of scientists must be engaged students.
The data presented in Figure 3 shows that 23.7% of students’ approach answering questions about heat and temperature using logic or common sense, 11.2% of students think/practice/apply information, and 15.2% rely on using prior experience. The knowledge that students already possess about the world is vast. Without the framework they have built for the physical world, they would be unable to function. People forget unrelated factoids as soon as they are no longer thought to be useful, therefore the only way to develop true scientific literacy is through the collection of ideas rather than knowledge (Harris, 1997). However, it is usual for school textbooks to omit or convey many aspects of science and the nature of science insufficiently.
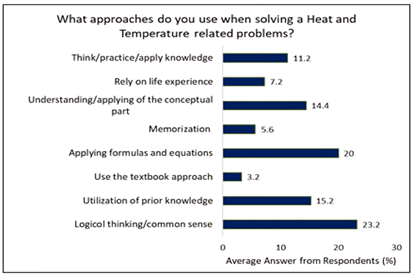
Additionally, 20% of students use formulas and equations to solve questions about heat and temperature. 14.4% try to comprehend and use conceptual reasoning. 7.2% of students also draw on their real-world experiences. When their current beliefs can be reconciled with what they learn, learning new information is not a challenge for kids. Many of the activities do involve forming connections between what they already know and new information (Hewson & Hewson, 1992, p. 61). This approach to heat and temperature concepts might be risky because it can result in erroneous beliefs. Through contact with their physical and social environment, including the cultural usage of imprecise language, alternative views may develop (Yeo & Zadnik, 2001). Additionally, textbooks fail to appropriately reflect the nature of science, notably the uncertain nature of scientific theories, according to Gegios et al. (2017). As a result, students who memorize formulas and concepts without comprehending the underlying principles may find it difficult to apply them in various situations.
According to Figure 4, it is clear that 60.78% of the students correctly answered question 3, while 31.07% and 33.98% of the students correctly answered question 1 and question 2, respectively. It is noteworthy that respondents who correctly responded to all three questions exhibited a confidence level that ranged from moderate to high. This is consistent with the DK effect that students do not know what they do not know which only confirms their weak metacognitive skills (Kruger & Dunning, 1999).
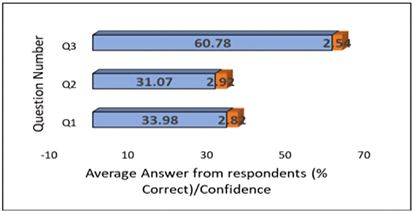
College seniors had the highest percentage of accurate answers across all questions, as shown by Figure 5. For each question, the accuracy differed among respondents from various academic years. For question 1, the accuracy rate for freshmen was 31.11%, for sophomores it was 37.78%, for juniors it was 29.07%, and for seniors it was 38.89%. Freshmen scored 15.56% on question 2, sophomores scored 35.56%, juniors scored 27.03%, and seniors scored 43.4%. Freshmen scored 62.2%, sophomores 57.79%, juniors 63.16%, and seniors 66.04% on question 3.
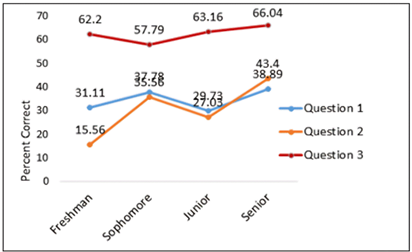
CONCLUSIONS
The study’s findings show that students generally lack a deep conceptual understanding of heat and temperature concepts. This is corroborated by earlier studies in science education that point to student misunderstanding, particularly with regard to grasping the definition of thermal equilibrium. The study also shows that students comprehend the value of having a thorough grasp of heat and temperature for resolving associated issues, which is consistent with previous work. The study of open-ended questions also reveals that students have trouble understanding the concepts of heat and temperature as well as converting between different temperature units. This is in line with earlier research that found difficulties separating heat from temperature in vast and intensive frameworks. For some students, remembering the formulas needed to solve heat and temperature-related problems can be difficult.
The study data suggest that students use a variety of tactics to answer questions about heat and temperature, including logical reasoning, the use of mathematics and equations, conceptual reasoning, and reliance on personal experience. However, the emphasis on memorizing and the absence of a comprehension of the guiding principles may limit students’ capacity to apply ideas in many situations and get a thorough understanding of science. In addition, the study shows that seniors, as opposed to freshmen, sophomores, and juniors, tend to have higher accuracy rates when responding to questions about heat and temperature. This shows that students’ comprehension of heat and temperature concepts increases as they advance through their scholastic years.
Overall, the study’s findings shed light on the difficulties students have learning about heat and temperature concepts and emphasize the necessity for efficient teaching methods that encourage comprehension rather than memorization. These results should be considered by educators and curriculum writers when creating science teaching methods and instructional materials that encourage students to learn science in a meaningful way and to become scientifically literate.
Author contributions: IIS: conceptualization, supervision; OF: investigation, writing - original draft; SA: writing - review and editing. All authors have agreed with the results and conclusions.
Funding: No funding source is reported for this study.
Ethical statement: The authors stated that the study was approved by the administration of the survey and data gathering were approved by the City College of New York Internal Review Board on 12 June 2022 (Approval code: 2022-0780-CCNY). Written informed consents were obtained from the participants.
Declaration of interest: No conflict of interest is declared by the authors.
Data sharing statement: Data supporting the findings and conclusions are available upon request from the corresponding author.
References
- Ahtee, M., & Varjola, I. (1998). Students’ understanding of chemical reactions. International Journal of Science Education, 20(3), 305-316. https://doi.org/10.1080/0950069980200304
- Alwan, A. (2011). Misconception of heat and temperature among physics students. Procedia-Social and Behavioral Sciences, 12, 600-614. https://doi.org/10.1016/j.sbspro.2011.02.074
- Ashenafi, M. M. (2017). Peer-assessment in higher education–Twenty-first century practices, challenges and the way forward. Assessment and Evaluation in Higher Education, 42(2), 226-251. https://doi.org/10.1080/02602938.2015.1100711
- Ausubel, D. P. (1968). Educational psychology: A cognitive view. Holt, Rinehart and Winston.
- Boujaoude, S. B. (1992). The relationship between students’ learning strategies and techniques in their understanding during a high school chemistry course. Journal of Research in Science Teaching, 29, 687-699. https://doi.org/10.1002/tea.3660290706
- Braaten, M., & Windschitl, M. (2011). Working toward a stronger conceptualization of scientific explanation for science education. Science Education, 95(4), 639-669. https://doi.org/10.1002/sce.20449
- Chu, H. E., Treagust, D. F., Yeo, S., & Zadnik, M. (2012). Evaluation of students’ understanding of thermal concepts in everyday contexts. International Journal of Science Education, 34(10), 1509-1534. https://doi.org/10.1080/09500693.2012.657714
- Chua, E., & Maloles, F. (2020). Activating strategies in teaching physical science: Direction towards enhanced students’ critical thinking skills. International Multidisciplinary Research Journal, 2(3), 174-181. https://doi.org/10.54476/iimrj348
- Colburn, A. (2009). Understanding heat and temperature. Science Teacher, 76(1), Article 10.
- Farooq, A., Haq, I. U., Haq, K. U., Bashir, A., & Riaz, H. (2021). Comparative study on the digital game and computer simulation to curtail Student’s misconception about heat & temperature. European Journal of Physics Education, 12(2), 1-10.
- Gabel, D. (1999). Improving teaching and learning through chemistry education research: A look to the future. Journal of Chemical Education, 76(4), 548-554. https://doi.org/10.1021/ed076p548
- Garnett, P. S., & Treagust, D. (1992). Conceptual difficulties experienced by senior high school students of electrochemistry, electric circuits and oxidation-reduction reactions. Journal of Research in Science Teaching, 29(2), 121-142. https://doi.org/10.1002/tea.3660290204
- Gegios, T, Salta, K, & Koinis, S. (2017). Investigating high-school chemical kinetics: The Greek chemistry textbook and students' difficulties. Chemistry Education Research and Practice, 18, 151-168. https://doi.org/10.1039/C6RP00192K
- Griffiths, A. K., & Preston, K. R. (1992). Conceptual difficulties experienced by senior high school students in electrochemistry: Electrochemical (galvanic) and electrolytic cells. Journal of Research in Science Teaching, 29, 1079-1099. https://doi.org/10.1002/tea.3660291006
- Hanrahan, S. J., & Isaacs, G. (2001). Assessing self- and peer-assessment: The students’ views. Higher Education Research & Development, 20(1), 53-70. https://doi.org/10.1080/07294360123776
- Harris, H. (1997). The chemistry classroom: Formulas for successful teaching (Herron, J. Dudley). Journal of Chemical Education, 74(10), 1167-1168. https://doi.org/10.1021/ed074p1167.3
- Hernandez, P., & Edinson, E. (2021). Use of analogies in science education, a systematic mapping study. arXiv. https://doi.org/10.48550/arXiv.2108.00849
- Hesse, J. J., & Anderson, C. W. (1992). Students’ conceptions of chemical change. Journal of Research in Science Teaching, 29, 277-299. https://doi.org/10.1002/tea.3660290307
- Hewitt, P. G. (2006). Conceptual physics. Addison-Wesley.
- Horton, A. C. (2007). Student alternative conceptions in chemistry. http://www.scribd.com/doc/52664732/student-alternative-conceptions-in-chemistry
- Jasien, P. G., & Oberem, G. E. (2002). Understanding of elementary concepts in heat and temperature among college students and K-12 teachers. Journal of Chemical Education, 79(7), 889-895. https://doi.org/10.1021/ed079p889
- Kesidou, S., Duit, R., & Glynn, S. M. (1995). Conceptual understanding in physics: Students’ understanding of heat. In S. M. Glynn, & R. Duit (Eds.), Learning science in the schools: Research reforming practice (pp. 179-198). Routledge.
- Kramarová, L., & Proksa, M., (2020). Students’ preconceptions about heat, temperature and energy. Chemistry Didactics Ecology Metrology, 25(1), 79-91. https://doi.org/10.2478/cdem-2020-0005
- Kruger, J., & Dunning D. (1999). Unskilled and unaware of it: How difficulties in recognizing one’s own incompetence lead inflated self-assessment, Journal of Personality and Social Psychology, 77(6), 1121-1134.
- Lewis, E., & Linn, M. C. (1994). Heat, energy and temperature concepts of adolescents, adults and experts: Implications for curriculum development. Journal of Research in Science Teaching, 31(6), 657-77. https://doi.org/10.1002/tea.3660310607
- Lythcott, J. (1990), Problem solving and the requisite knowledge of chemistry. Journal of Chemical Education, 67, 248-252. https://doi.org/10.1021/ed067p248
- Magnus, J. R., & Peresetsky, A. A. (2022). A statistical explanation of the Dunning-Kruger effect. Frontiers in Physhology, 13. https://doi.org/10.3389/fpsyg.2022.840180
- Mason, L., & Zaccoletti, S. (2020). Inhibition and conceptual learning in Science: A review of studies. Educational Psychology Review, 33(1), 181-212. https://doi.org/10.1007/s10648-020-09529-x
- McCain, K. (2015). Explanation and the nature of scientific knowledge. Science & Education, 24(7-8), 827-854. https://doi.org/10.1007/s11191-015-9775-5
- National Research Council, Board on Science Education, National Committee on Science Education Standards, & Assessment. (1995). National science education standards. National Academies Press.
- Novick, S., & Nussbaum, J. (1978). Junior high school students’ understanding of the particulate nature of matter, an interview study. Science Education, 62, 273-281. https://doi.org/10.1002/sce.3730620303
- Papinczak, T., Young, L., & Groves, M. (2007). Peer assessment in problem-based learning: A qualitative study. Advances in Health Sciences Education, 12(2), 169-186. https://doi.org/10.1007/s10459-005-5046-6
- Permata, D., Wijayanti, P., & Masriyah. (2019). Students’ misconceptions on the algebraic prerequisites concept: Causative factors and alternative solutions. Journal of Physics: Conference Series, 1265, 012005. https://doi.org/10.1088/1742-6596/1265/1/012005
- Peterson, R. F., & Treagust, D. F. (1989). Grade-12 students’ misconceptions of covalent bonding. Journal of Chemical Education, 66, 459-460. https://doi.org/10.1021/ed066p459
- Schmidt, H. J. (1997). Students’ misconceptions–Looking for a pattern. Science Education, 81(2), 123-135. https://doi.org/10.1002/(SICI)1098-237X(199704)81:2<123::AIDSCE1>3.0.CO;2-H
- Schullery, E. S. (1979). Formal operational–Where is it really needed? Journal of Chemical Education, 56(11), Article 768. https://doi.org/10.1021/ed056p768.1
- Shulman, L. S. (1986). Those who understand: Knowledge growth in teaching. Educational Researcher, 15(2), 4-14. https://doi.org/10.3102/0013189X015002004
- Taber, S. K. (2019). The nature of the chemical concept: Re-constructing chemical knowledge in teaching and learning. Royal Society of Chemistry.
- Thomas, P. L., & Schwenz, R. W. (1998). College physical chemistry students’ conceptions of equilibrium and fundamental thermodynamics. Journal of Research in Science Teaching, 35(10), 1151-1160. https://doi.org/10.1002/(SICI)1098-2736(199812)35:10<1151::AID-TEA6>3.0.CO;2-K
- Thomaz, M., Valente, M. C., Maliquias, I. M., & Aritanes, M. (1995). An attempt to overcome alternative conceptions related to heat and temperature. Physics Education, 30(1), 19-36. https://doi.org/10.1088/0031-9120/30/1/004
- Tümay, H. (2016). Reconsidering learning difficulties and misconceptions in chemistry: Emergence in chemistry and its implications for chemical education. Chemistry Education Research and Practice, 17(2), 229-245. https://doi.org/10.1039/C6RP00008H
- Yeo, S., & Zadnik, M. (2001). Introductory thermal concept evaluation: Assessing students' understanding. The Physics Teacher, 39, 496-504. https://doi.org/10.1119/1.1424603
- Zamora-Polo, Corrales-Serrano, M., Sánchez-Martín, J., & Espejo-Antúnez, L. (2019). Nonscientific university students training in general science using an active-learning merged pedagogy: Gamification in a flipped classroom. Education Sciences, 9(4), 297-315. https://doi.org/10.3390/educsci9040297
How to cite this article
APA
Salame, I. I., Fadipe, O., & Akter, S. (2025). Examining difficulties, challenges, and alternative conceptions students exhibit while learning about heat and temperature concepts. Interdisciplinary Journal of Environmental and Science Education, 21(2), e2510. https://doi.org/10.29333/ijese/15997
Vancouver
Salame II, Fadipe O, Akter S. Examining difficulties, challenges, and alternative conceptions students exhibit while learning about heat and temperature concepts. INTERDISCIP J ENV SCI ED. 2025;21(2):e2510. https://doi.org/10.29333/ijese/15997
AMA
Salame II, Fadipe O, Akter S. Examining difficulties, challenges, and alternative conceptions students exhibit while learning about heat and temperature concepts. INTERDISCIP J ENV SCI ED. 2025;21(2), e2510. https://doi.org/10.29333/ijese/15997
Chicago
Salame, Issa I., Oluwamuyiwa Fadipe, and Samiha Akter. "Examining difficulties, challenges, and alternative conceptions students exhibit while learning about heat and temperature concepts". Interdisciplinary Journal of Environmental and Science Education 2025 21 no. 2 (2025): e2510. https://doi.org/10.29333/ijese/15997
Harvard
Salame, I. I., Fadipe, O., and Akter, S. (2025). Examining difficulties, challenges, and alternative conceptions students exhibit while learning about heat and temperature concepts. Interdisciplinary Journal of Environmental and Science Education, 21(2), e2510. https://doi.org/10.29333/ijese/15997
MLA
Salame, Issa I. et al. "Examining difficulties, challenges, and alternative conceptions students exhibit while learning about heat and temperature concepts". Interdisciplinary Journal of Environmental and Science Education, vol. 21, no. 2, 2025, e2510. https://doi.org/10.29333/ijese/15997