Abstract
The present study aims to design an instruction that engages nature of science (NOS) and nature of scientific inquiry (NOSI) learning objectives with the teaching and learning of important historical experiments taught around the world and presented in most biology textbooks such as those by Griffith and by Avery, MacLeod, and McCarty. The design process involves decisions about which NOS and NOSI aspects to teach and decisions about how to teach. With respect to the latter decisions, our preference is an explicit/reflective perspective used in an overarching inquiry-based science teaching and history of science instructional context. The proposed course is going to provide high school students meaningful and practical inquiry-based experiences through the use of simulated experiments and would help their teachers (a) illuminate several procedural aspects of the taught experiments (b) internalize the importance of teaching NOS and NOSI aspects (c) acquire an integrative understanding of the process of scientific inquiry and the product of that inquiry, namely scientific knowledge, and (d) portray science less as a body of knowledge and more as a creative process involving human and non-human actors. The potential benefits of our proposed instruction for learners’ scientific literacy are also discussed.
Keywords
License
This is an open access article distributed under the Creative Commons Attribution License which permits unrestricted use, distribution, and reproduction in any medium, provided the original work is properly cited.
Article Type: Research Article
INTERDISCIP J ENV SCI ED, Volume 20, Issue 4, 2024, Article No: e2421
https://doi.org/10.29333/ijese/15522
Publication date: 24 Oct 2024
Article Views: 581
Article Downloads: 412
Open Access HTML Content Download XML References How to cite this articleHTML Content
INTRODUCTION
Scientific literacy is a major goal of science education throughout the world and consists of four major components (Kampourakis, 2016; Lederman et al., 2013). To be scientifically literate, learners must have not only conceptual and procedural knowledge, but also knowledge about the nature of science (NOS) and knowledge about the nature of scientific inquiry (NOSΙ).
Knowledge about NOS is defined as knowledge about the epistemological underpinnings of science (Gess-Newsome, 2002) and addresses issues that characterize science as a special form of constructing knowledge (García-Carmona & Acevedo-Díaz, 2017). The response to the question of what to teach about NOS at K-12 settings is not simple and remains the subject of a permanent debate within the international science education community (Allchin, 2011; Clough, 2018; Dagher & Erduran, 2016; Kampourakis, 2016; Schizas et al., 2016; Wallace, 2017). However , one response also called as the consensus view has dominated the international scene over the last two decades (Garcia-Carmona, 2021) and includes some epistemic (i.e., cognitive and rational aspects related to both scientific knowledge and the processes and methods of science; Aragón-Méndez et al., 2019) and non-epistemic (i.e., contextual, social and psychological aspects that relate to science and scientists; Garcia-Carmona, 2021) aspects of science, such as (e.g., Lederman et al., 2002; Mesci & Schwartz, 2017):
-
scientific knowledge is empirical, tentative, partly the product of human imagination and creativity, theory-laden, subjective, embedded in social and cultural contexts and not obtained through a universal step-by-step scientific method, and
-
scientific laws are descriptive statements about the discerned patterns of natural phenomena and differ from scientific theories, which are inferred explanations of those phenomena.
Additionally, knowledge about scientific inquiry (SI) extends beyond procedural knowledge and the mere know-how understanding of process skills such as observing, inferring, classifying, predicting, measuring, questioning, interpreting, and analyzing data. NOSI knowledge refers to understanding SI and involves an understanding of SI aspects related to what/why scientists work as they do and how knowledge is accepted within the scientific community (Leblebicioglu et al., 2020; Lederman, 2018). Furthermore, while there are no consensus lists of NOSI (Mesci et al., 2020), as it is the case with NOS, most contemporary NOSI researchers consider eight essential NOSI aspects as suitable for teaching K-12 grades (Lederman et al., 2014). These aspects have also been identified by the national science education standards (NGSS Lead States, 2013) and can be defined, as follows:
-
all scientific investigations begin with a question and do not necessarily test a hypothesis,
-
there is no single set or sequence of phases followed in all investigations,
-
inquiry procedures are guided through a question,
-
all scientists performing the same procedures may not get the same results,
-
inquiry procedures can influence results,
-
research conclusions must be consistent with the data collected,
-
scientific data are not the same as scientific evidence, and
-
explanations are developed through a combination of collected data and what is already known.
While research on the teaching and learning of NOS has a long history and has evolved in recent decades, there is little research on the teaching and learning of NOSI (Lederman, 2018) due to the lack of valid and reliable assessment instruments to measure learners’ understandings of SI (Mesci et al., 2020). The recent publication of the views of scientific inquiry (Schwartz et al., 2008) instrument and the views about scientific inquiry (VASI) (Lederman et al., 2014) instrument has accelerated research on students and teachers understanding of NOSI and demonstrated that, similar to research on understandings of NOS, students and teachers typically hold naive views (Leblebicioglu et al., 2020; Lederman et al., 2019; Penn et al., 2021; Senler, 2015; Yang et al., 2017). For example, the most recent international study (Lederman et al., 2021) used the VASI instrument to collect data on what exiting high school students in 32 countries and regions, spanning six continents learned about NOSI. The results were not considered satisfactory because they showed that the worldwide frequencies of informed understandings for the aspects of NOSI were all below 45%.
NOSI and NOS are often used as synonymous terms and research on NOSI has often been conflated with research on NOS under a more general “learners’ understandings of science” (Schwartz et al., 2008; Wacker et al., 2022). Nevertheless, contemporary scholars agree that NOS knowledge covers the features of scientific knowledge, whereas NOSI knowledge covers the characteristics of the SI through which scientific knowledge is constructed and accepted (Schwartz et al., 2008), and through this distinction they conduct separate research on NOS and NOSI. However, NOSI and NOS are interdependent and a didactic combination of the epistemic aspects of NOSI with the epistemic and especially with the non-epistemic aspects of NOS could prove beneficial to students and teachers. The history, philosophy and sociology of science demonstrate the important influence of multiple non-epistemic aspects on the development of science (García-Carmona, 2021) and their combination with the epistemic aspects of NOSI in teaching proposals would help learners acquire an integrative understanding of the process of SI and the product of that inquiry, namely scientific knowledge. This integration can ensure both an adequate enactment of SI in educational settings and an understanding of science less as a body of knowledge and more as what really is: a creative process addressed by human and non-human actors (Stamou, 2012).
Because of this beneficial impact on learners, science education researchers highlight the need for teachers to internalize the importance of aspects related to the NOS and SI and recommend the enrichment of literature with research on the integration of NOS and NOSI with other curriculum content. This integration in teaching proposals could enhance students’ understanding of conceptual and procedural knowledge and could motivate science teachers to deal with NOS and NOSI in their classes without having to greatly alter the attention they give to other science content (Bell et al., 2012; Garcia-Carmona, 2021). This is particularly important for science teachers who encounter difficulties in transferring their understanding of NOSI and NOS into their teaching practices (Mesci 2016; Mesci et al., 2020) and for countries where explicit allusions to NOS and NOSI are minimal in school science curricula.
Driven by this research call for developing teachers’ valuing of NOS and NOSI, the present study aims to design an instruction that targets high school students with a biology background and combines the teaching of curriculum content with NOSI and NOS aspects, especially those that are difficult for students and teachers to understand. The teachable curriculum content concerns important historical experiments taught around the world and presented in most biology textbooks such as those by Griffith and by Avery, McLeod, and McCarty (Hoefnagels, 2015; Mader, 2009; Mader & Windelspecht, 2019; Raven et al., 2017; Sadava et al., 2016; Solomon et al., 2011; Urry et al., 2017). The NOSI and NOS aspects, which will accompany the curriculum content in the form of complementary learning objectives, concern certain aspects of NOSI that create difficulties in learners’ understanding, such as the aspects of “scientific investigations begin with a question “(Mesci, 2016), ‘inquiry procedures are guided by the question asked’ (Leblebicioğlu et al., 2017) and ‘research conclusions must be consistent with the data collected’ (Mesci et al., 2020), as well as non-epistemic aspects of NOS. Aside from the problems learners encounter in understanding non-epistemic NOS items (García-Carmona, 2021), the way Griffith’s experiments and those of Avery, MacLeod, and McCarty are commonly presented in biology textbooks obscures many aspects of their historical unfolding and likely reinforces misconceptions among students worldwide about what science is. For example, a close look at the 12th grade Greek biology textbook (Aleporou-Μarinou et al., 1999) from which we’ll draw the material to design our instruction reveals that science (biology) is presented as an objective, non-creative and timeless social practice that accumulates empirical facts. Therefore, in parallel with the design of our teaching proposal, we’ll didactically transform the relevant knowledge presented in the textbook discussed above so that we can formulate and achieve the desired learning objectives.
As for the structure of this paper, we start by setting out the goals of our study. Subsequently, we present the text of the textbook to be didactically transformed and the design features of our teaching proposal. Next, we present our teaching proposal, which consists of three sections. The first section introduces students to the historical context in which Griffith’s experiments and those of Avery, MacLeod, and McCarty were conducted. The second and third sections refer to the experimental manipulations of Griffith and of Avery, MacLeod, and McCarty, respectively.
Goals of the Study
The proposed instruction aims at enhancing the learning experience of both high school students in Greece, who are taught biology through the compulsory 12th grade biology textbook and their biology specialists teachers by integrating specific learning objectives related to the procedural knowledge in question with objectives related to specific knowledge about NOSI and NOS. These objectives encompass the following key aspects:
-
Understand how scientific inquiries start: Make students aware that scientific investigations typically begin with a question. Emphasize that while testing a hypothesis is a frequent part of scientific inquiries, it is not the starting point.
-
Formulate scientific questions and understand their role: Enable students to formulate scientific questions similar to those that Griffith asked during his experimental manipulations. Emphasize the necessity of aligning research methods with the specific scientific questions posed.
-
Comprehend scientific hypotheses: Familiarize students with the key characteristics of scientific hypotheses, enhancing their ability to understand the role of hypotheses in science studies.
-
Design experimental manipulations: Develop the ability to propose experimental manipulations that can be used to test and support previously formulated scientific hypotheses.
-
Interpret experimental results: Develop students’ abilities to provide explanations for experimental results and formulate scientific hypotheses based on empirical findings.
-
Evaluate research conclusions: Facilitate the understanding of when experimental results can support or refute a scientific hypothesis and highlight the importance of data consistency and interpretation in drawing valid conclusions.
-
Appreciate the creativity in science: Help students recognize the creativity involved in scientific endeavors and understand that science is not just a collection of facts but a dynamic process of inquiry and discovery.
-
Acknowledge the socio-cultural context of science: Foster an appreciation of the socio-cultural context in which science operates, recognizing that scientific knowledge is influenced by social, cultural, and internal factors within the scientific community.
Given that our study is not empirical research but rather a theoretical proposal for an instructional design, the methodological approach centers on elaborating and structuring an instructional framework aimed at teaching aspects of NOS and NOSI within the context of historical biology experiments. The primary question thus is how an instructional approach can be effectively designed to serve and accomplish the mentioned above learning objectives.
The Experiments of Giffith and of Avery, MacLeod, and McCarty Presented in the Greek Biology Textbook
Giffith’s experiments, as well as those of Avery, MacLeod, and McCarty, are part of the Greek biology curriculum in high schools. High school students are introduced to these experiments in chapter 1 of the 12th grade biology textbook written by experienced biology teachers and published by the Greek Ministry of Education, Lifelong Learning and Religious Affairs (Aleporou-Μarinou et al., 1999). This chapter is entitled “the genetic material” and covers topics such as the chemical constitution and structure of DNA and the number and shape of chromosomes in humans and prokaryotic organisms.
The multimodal text of the textbook is the following:
“In 1928, Griffith (1928) studied two strains of the bacterium Diplococcus pneumoniae that differ morphologically in the presence or absence of an outer capsule that protects them from an animal’s immune system. The strain with the protective capsule formed smooth colonies and was pathogenic, i.e., it killed the mice it infected, while the strain without the protective capsule formed rough colonies and was nonpathogenic.
Griffith used high temperatures to kill the smooth bacteria and infected mice with them. As a result, mice remained alive. However, when he mixed dead smooth bacteria with live rough bacteria and used the mixture to infect mice, they died. Live smooth bacteria were found in the blood of the dead mice. Griffith concluded that some rough bacteria had turned into smooth pathogens after interacting with the dead smooth bacteria, but he could not give a satisfactory answer as to how this happened (see image).
The answer was given in 1944 when Avery, MacLeod, and McCarty repeated Griffith’s experiments in vitro. The researchers separated the chemical components of the dead smooth bacteria into carbohydrates, proteins, lipids, RNA, DNA, etc. and tested which of these had the ability of transformation. They found that the component that caused the transformation of the rough bacteria into smooth bacteria was DNA.
The definitive confirmation that DNA is the genetic material came in 1952 with the classic experiments of Hershey and Chase who studied the life cycle of the bacterium T2 …”
INSTRUCTIONAL DESIGN METHODOLOGY
Our study adopts a structured teaching methodology that is informed by the latest educational research on inquiry-based science teaching (IBST), NOS, and NOSI. The methodology comprises the following key components:
-
Development of an explicit/reflective guided inquiry approach
NOSI researchers have not devised a specific method for teaching NOSI and drawing on analogous findings from research on NOS recommend the use of explicit/reflective teaching approaches (Abd-El-Khalick, 2012; Mesci & Schwartz, 2017; Mesci et al., 2020). In such approaches, learners are more or less guided to explore and construct the targeted NOSI definitions on their own, but at some point, they are provided with information and instructional support that can help them revise or refine their own ideas and further elaborate on the targeted NOSI definitions (Vorholzer et al., 2020). The notion of “explicit” differs from specifications of explicit that merely focus on whether or not learners have been told NOS or NOSI definitions and emphasizes the importance of supporting conceptual understanding through well-planned instruction and focused practice. The notion of “reflective” calls for instructional decisions that can help learners reflect on their own and others’ understandings of aspects of NOS or NOSI and discuss their conceptions in the learning activities in which they are engaged.
NOS researchers have developed different strategies for teaching NOS through the use of explicit/reflective teaching approaches without favoring one strategy over another (Khishfe & Lederman, 2007). The teaching of NOS can be planned as content-dependent contextualized from other school science content (e.g., Michel & Neumann, 2016; Schizas et al., 2022), as independent content de-contextualized from that other content (e.g., Lederman & Abd-El-Khalick, 1998), or as a combination of the two strategies (e.g., Akerson & Donnelly, 2010). NOSI researchers on the other hand suggest the use of IBST as an explicit/reflective context for addressing aspects of NOSI (BSCS, 2009; Capps & Crawford, 2013). In support of this suggestion, the results of recent research studies show that students and teachers improve their understanding of NOSI aspects when they experience these aspects explicitly and reflectively in contextualized inquiry-based learning activities (Hrisa & Psillos, 2022; Leblebicioğlu et al., 2017).
Regarding thus our choices referring to how to teach, our preference is an explicit/reflective perspective used in an overarching IBST instructional context. The historical experiments conducted by Griffith and Avery, MacLeod, and McCarty, are used to contextualize NOS and NOSI and serve as the primary narrative line for instruction. This line will be based on how the Greek biology textbook (Aleporou-Μarinou et al., 1999) presents these experiments and will guide us in defining NOSI and non-epistemic NOS learning objectives whose source is history of science (HOS).
-
Integration of History of Science
Apart from integrating key historical experiments to provide context and depth to the NOSI items being taught, our instructional design leverages HOS to highlight non-epistemic aspects of NOS, such as the social and cultural aspects of SI. HOS compared to contemporary epistemological analyses of science can make clearer how scientists dealt with the challenges of their research, what role the scientific community played in the construction and acceptance of scientific ideas, what influence the social, political, economic and cultural contexts had and other non-epistemic aspects (García-Carmona, 2021).
-
Utilization of Simulated Experiments
Embedded within an IBST and HOS instructional framework, the proposed instruction employs simulated experiments to replicate the key historical experiments in a virtual and interactive environment. This environment allows students to engage in inquiry-based practices that are easily accessible and pedagogically effective.
Research on the pedagogical exploitation of simulated experiments shows that virtual experimentation is more effective than other traditional methods in teaching science concepts and addressing a variety of science process skills including visualization, problem-solving, identification, classification, data interpretation, and experimental design (Rutten et al., 2012). Simulated experiments are powerful tools for enhancing learning because they allow learners to explore physical or biological situations that may be impossible, too expensive, difficult, or time-consuming to accomplish with actual laboratory or real-life experiences (Akpan, 2001; Lee et al., 2002; Psillos, 2023; Thomas & Vo, 2021). In addition, they effectively promote the development and linkage of conceptual knowledge to scientific practices that underlie SI.
An open-access online simulator found at the web address http://www.cheminfo.org/Demo/Griffith_experiment/index.html will be used in the teaching of Griffith’s experiment. The interface of this simulator represents a virtual laboratory consisting of 6 windows (Figure 1). The first central window in the left corner contains the necessary materials and tools for students to interact with when conducting Griffith’s experiment. Two windows show the two strains of bacteria as they appear in the light of the microscope, and two other windows represent the possible actions and the performed actions to which students can proceed when they use the materials and tools. There is also a window that depicts what happens to the mice when certain experimental choices are made.
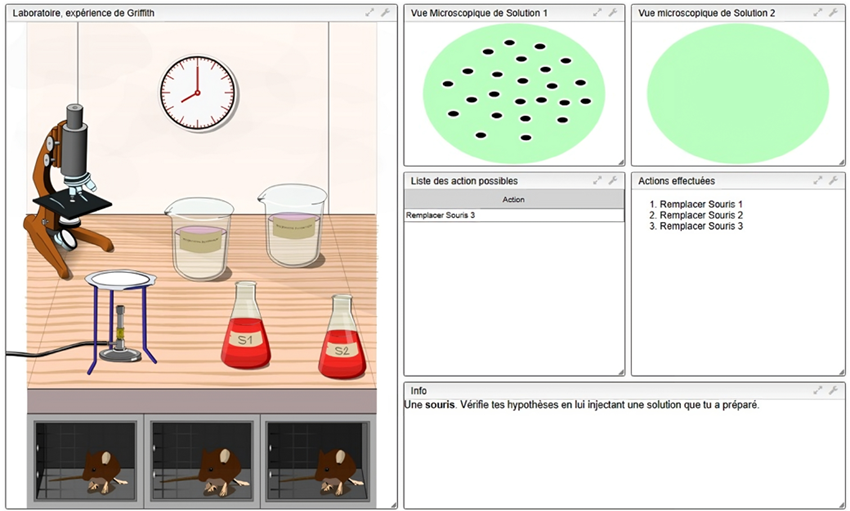
-
Combination of Engaging Activities
The proposed instructional approach prioritizes a student-centered learning experience and incorporates a range of activities designed to facilitate the learning of NOSI and NOS items through inquiry and reflection. These include:
-
Guided inquiry tasks: Structured activities on specially designed worksheets that guide students through the exploration of NOSI and NOS items using historical and simulated experiments.
-
Reflective writing: Opportunities for students to articulate their understanding and reflect on the inquiry process.
-
Group discussions: Collaborative learning sessions where students share insights and challenge each other’s ideas, fostering a deeper engagement with the material.
-
Contextualized learning: Lessons are designed to link NOSI and NOS items with students’ prior knowledge and real-world scientific practices, enhancing the relevance and applicability of the instruction.
These activities are designed to be both engaging and educational, offering multiple pathways for students with diverse learning styles to explore and understand NOSI and NOS.
INTEGRATING NOSI WITH NOS: A SCENARIO
Following the design theses and decisions discussed above, we present and analyze a teaching scenario that integrates NOSI aspects with procedural and NOS knowledge. This scenario, presented below, is divided into three main phases, and planned for a duration of three hours.
1st Phase: Introducing Students to the Historical Context in Which the Experiments of Griffith and of Avery, Macleod, and McCarty Were Conducted
Initiating the session, the teacher immerses students in the historical milieu that set the stage for experiments validating DNA as genetic material. This includes Griffith’s 1928 experiment and the collaborative work of Avery, MacLeod, and McCarty in 1944.
The teacher informs students about what scientists knew about DNA and proteins at that time, what they did not know, and what hypotheses they could come up with. (S)he discusses with students that
-
scientists in the first decades of the 20th century knew the chemical composition of DNA and proteins but did not know which of the two chemicals was ultimately the genetic material
-
lacking contemporary research data, scientists leaned toward proteins as the likely genetic material.
The first learning objective [L.O.1] centers on comprehending the historical context of Griffith’s experiment. To achieve this, the teacher guides students through collaborative thinking, prompting discussions on the hypotheses scientists might have formulated. Working in teams, students tackle the initial worksheet with three pertinent questions (Table 1).
Table 1. 1st worksheet
|
The first question (1a) probes what led scientists to support the hypothesis that proteins are the genetic material. Featuring a multiple-choice format with two tiers, it serves to instill a basic grasp of scientific hypotheses. The subsequent learning objective [(NOSI) L.O.2] underscores that scientific hypotheses are affirmative responses to research questions, emerging from logical reasoning and interpretations of existing scientific data.
Acknowledging the potential challenges in students’ comprehension of scientific hypotheses, the teacher addresses common misconceptions, such as perceiving a scientific hypothesis as an unsupported speculation or an educated guess (McComas, 1996). The teacher explicitly clarifies that the hypothesis proposing proteins as the genetic material originates from thoughtful reflection on prior knowledge and existing scientific data pertaining to the chemical composition of DNA and proteins. This clarification serves to underscore the hypothesis’s informed and evidence-based foundation.
Moving to the second question (2), students are tasked with formulating a complete argument using arrows in the homomorphic form of mechanistic reasoning. This structure aims to help students avoid logical gaps in the formulation of their arguments (Schizas et al., 2022). The first arrow focuses on why proteins exhibit greater diversity than nucleic acids, a straightforward task given the information available. The second arrow poses a more challenging question: why scientists believe that proteins, and not nucleic acids, constitute the genetic material due to their greater diversity. In case of difficulties, the teacher intervenes, using the third question (3) as a scaffold. This question, tied to a familiar practical problem, guides students in finding analogies to address question 2 comprehensively.
2nd Phase: Teaching Griffith’s Experiment
In this phase, the third learning objective [L.O.3] is for students to delve into Griffith’s scientific background and the motivations behind his famous 1928 experiment. The teacher enlightens students about Griffith’s primary focus on the epidemiology of infectious diseases, particularly his endeavors to discover a cure for pneumonia caused by diplococcus pneumoniae. Notably, Griffith operated in an era when the profound knowledge about vaccines, commonplace today, was absent.
Subsequently, the fourth learning objective [L.O.4] aims at equipping students with a comprehensive understanding of the procedural aspects of Griffith’s first and second experimental manipulations. Before immersing students in a simulated experiment, they tackle a second structured worksheet. Questions within this worksheet prompt students to consider the approach an immunobiologist might take in finding a cure for a contagious disease like pneumonia, emphasizing the connection to vaccine knowledge and the essential factors for vaccine development.
The teacher informs students that Griffith used two strains of Diplococcus pneumoniae bacteria in his experiment and introduced these strains into mice. One strain possesses an outer capsule, shielding it from animal’s immune system and forming smooth colonies, while the second strain lacks a capsule, resulting in rough colonies.
Encouraging collaboration, students are asked to discuss with their classmates how Griffith introduced these bacteria into mice and predict the outcomes of such manipulations. After becoming familiar with the virtual environment, students are prompted to engage with and perform the simulated experiment. They are guided to figure out that the two strains of bacteria were introduced into the mice by injection and are helped by using the knowledge of which strain of bacteria has the protective capsule against animals’ immune system to predict that
-
the result of Griffith’s first manipulation was the death of the mice and
-
the result of Griffith’s second manipulation was the survival of the mice.
Students are then encouraged to engage in a metacognitive activity in which they reflect on why these experimental manipulations were performed. To help students overcome potential difficulties in answering these questions, the teacher needs to remind students that Griffith wanted to find a cure for pneumonia and discuss with them certain aspects of SI, specifically that a SI always begins with a question and does not necessarily test a hypothesis. This is the fifth learning objective [(NOSI) L.O.5]. To illustrate this objective, the teacher points out that Griffith conducted these experimental manipulations because he had specific research questions in mind, and then guides students to formulate those questions. Recalling Griffith’s goal, students could answer that Griffith wanted to find out if these different strains of bacteria were pathogenic.
Building on the understanding gained thus far, the sixth learning objective (L.O.6) focuses on the procedural intricacies of Griffith’s third experimental manipulation. The teacher discloses that Griffith introduced dead pathogenic bacteria–smooth bacteria with a protective capsule–into mice. Students, using the simulation, grapple with questions on
-
how Griffith caused the death of these bacteria,
-
why heating a solution containing living organisms leads to their demise, and
-
the outcomes and research question stemming from Griffith’s manipulation.
Students may encounter cognitive hurdles, particularly in responding to questions (b) and (c). Concerning question (b), students might struggle to recall prior knowledge from lower grades pertaining to “protein denaturation” and the crucial role of proteins in biological processes. For question (c), the risk is that modern insights in immunobiology could impede students from accurately addressing Griffith’s original research query. Students know that dead organisms do not cause disease, and this is ensured when conducting the simulation. Students are then informed that
-
this knowledge was not available to scientists in Griffith’s time and
-
the finding of this knowledge motivated Griffith to perform his third experimental manipulation.
To guide students towards uncovering Griffith’s research question, the teacher introduces a hypothetical scenario. Students are prompted to imagine a situation where scientists lack knowledge about the distinctions between the biological and chemical levels and observe that dead pathogenic bacteria elicit disease symptoms in mice. The teacher encourages students to contemplate what these scientists would attribute as the cause of the observed disease symptoms. Through discussion and engagement with the teacher, students gradually realize that Griffith’s research question centered on determining whether disease symptoms were instigated by chemical substances within microorganisms–whether alive or deceased–or by the collective vitality of these microorganisms and their actions (Griffith 1928).
The seventh learning objective delves into the procedural aspects of Griffith’s fourth experimental manipulation. The teacher unfolds the scenario where dead pathogenic bacteria (with protective capsules) are introduced into mice alongside live non-pathogenic bacteria (without protective capsules). Students are prompted to predict the possible outcome, guided by a series of questions:
-
Can dead pathogenic bacteria alone cause the death of mice?
-
Can living non-pathogenic bacteria alone cause the death of mice?
-
Can a mixture of both bacteria lead to the death of mice?
Following their predictions, students execute the simulation and find that the mice stay alive. Then, guided by their instructor, they allow more time for the mixture of both bacterial strains to interact. Upon rerunning the simulation, a surprising outcome emerges, challenging both prior Griffith’s findings and the students’ earlier expectations. While individual bacterium types fail to induce mortality, the combined mixture results in significant fatalities.
Griffith’s perplexing findings in the fourth experimental manipulation serve as a gateway to the eighth learning objective, where students are introduced to NOS knowledge. The teacher emphasizes that science is a human endeavor marked by unpredictability, requiring scientists like Griffith to employ imagination and creativity to elucidate and explain unexpected results.
Griffith’s situation may become an authentic illustration of scientific creativity, offering students a chance to conceptually-emotionally engage with this notion in school science (Kind & Kind, 2007). Channeling Griffith’s mindset, students are tasked with formulating the scientific hypothesis that could explain the enigmatic experimental outcome. This demands logical reasoning and imaginative thinking as students explore the notion of a concealed explanation emerging for the first time in the history of biology. Acknowledging the difficulty of this creative task, the teacher shares Griffith’s hypothesis: the heating-induced release of components from dead pathogenic bacteria, transformed live non-pathogenic bacteria into pathogens upon introduction into the culture.
Moving to the ninth learning objective (L.O.9), students delve into the procedural aspects of Griffith’s concluding experimental manipulation. Prior to prompting students to describe this manipulation, the teacher introduces another facet of scientific hypotheses, emphasizing their purpose (Keeley et al., 2008). The tenth learning objective [(NOSI) L.O.10] elucidates that scientific hypotheses are formulated to explain observations, such as discernible patterns in nature and serve as guides for researchers in designing experimental procedures that bolster their credibility.
The teacher then asks students to envision how they would either support or refute Griffith’s earlier scientific hypothesis. Drawing parallels with cases of human death where causes are determined through post-mortem examinations, the teacher directs students’ attention to the examination of the dead mouse tissue. Subsequently, students are prompted to specify the kinds of results related to the dead mouse tissue that could either confirm or contradict Griffith’s hypothesis. Emphasizing the need for evidence to support Griffith’s research conclusion, the teacher reveals that Griffith did detect live pathogenic bacteria in the mouse tissues, substantiating his hypothesis and delves into the eleventh learning objective [(NOSI) L.O.11]: research results must be consistent with the data collected.
3rd Phase: Teaching the Avery, MacLeod, and McCarty Experiment
The teacher initiates this phase by highlighting the common occurrence in scientific history where unexpected results, such as bacterial transformation in Griffith’s case, lead to significant discoveries. Acknowledging the gaps in Griffith’s knowledge, the teacher prompts students to reflect on what remained unknown during Griffith’s time, specifically regarding the components responsible for transforming non-pathogenic bacteria into pathogens—whether DNA or proteins.
Following this, the teacher introduces the pivotal work of Avery, MacLeod, and McCarty, emphasizing their attempt to answer the lingering question through a meticulous repetition of Griffith’s fourth experimental manipulation under “in vitro” conditions. An essential learning objective [(NOSI) L.O.12] here is to convey to students that the choice of research methods is intricately tied to the research question, underscoring the guiding influence of the latter.
The third learning activity unfolds, prompting students to engage with two worksheets that delve into the procedural aspects of Avery, MacLeod, and McCarty’s experiments. The thirteenth learning objective [L.O.13] is for students to comprehend the procedural intricacies of the first experimental manipulation by the aforementioned scientists. A series of guided questions helps students navigate this understanding:
-
In their “in vitro” experiment, what did Avery, MacLeod, and McCarty omit compared to Griffith? Where did they conduct their experiment?
-
Griffith confirmed bacterial transformation by finding live pathogenic bacteria in the tissues of dead mice. If dead pathogenic bacteria and live non-pathogenic bacteria are mixed in test tubes, what is anticipated to happen?
-
To discern whether proteins or DNA from dead pathogenic bacteria induce transformation in live nonpathogenic bacteria, what experimental steps need to be taken?
Recognizing that students might face challenges in answering the last question, the teacher employs scaffolding, presenting a hypothetical scenario involving patient treatment with a solution containing various chemicals (A, B, C, and D). By drawing parallels, students are encouraged to think critically about experimental design and deduction. The guiding questions are the following:
-
If you cannot answer the question, consider the following hypothetical problem: Suppose we have a solution containing chemicals A, B, C, and D. We give this solution to a large number of patients, and they are treated. How can I find out which substance was responsible for curing the patients? Can you answer now?
The teacher proceeds to detail Avery, MacLeod, and McCarty’s experiment, highlighting key steps such as
-
preparing a laboratory extract with dead pathogenic bacteria,
-
employing chemicals to eliminate lipids, carbohydrates, proteins, and RNA,
-
combining the extract with nonpathogenic bacteria, and
-
observing the transformation of the nonpathogenic strain into a pathogenic one.
Students are prompted to articulate the research question, formulate the scientific hypothesis tested, and assess whether the results support or refute the hypothesis. Subsequently, they are tasked with determining the sufficiency of this manipulation in establishing whether DNA, as opposed to proteins, serves as the genetic material.
To help students determine this sufficiency and elucidate the second experimental manipulation by Avery, MacLeod, and McCarty, the teacher employs a series of guiding questions (included on the last worksheet), such as the following:
-
Although Avery, MacLeod, and McCarty knew that the DNA of dead pathogenic bacteria transferred to living nonpathogenic bacteria and transformed them into pathogens, did they know that the proteins of dead pathogenic bacteria did not cause a similar transformation?
-
Do not they need this knowledge to decide whether DNA or proteins are the genetic material?
-
If this knowledge is necessary, what could they do to complete the first experimental design?
The fourteenth learning objective [L.O.14] involves comprehending the procedural aspects of Avery, MacLeod, and McCarty’s second manipulation. Here, students learn that the researchers selectively destroyed DNA with chemicals while leaving proteins intact. They are once again tasked with framing the research question and hypothesis, along with determining the congruence of the results with the hypothesis.
The discussion extends to Hershey and Chase’s bacteriophage T2 experiment, described in the textbooks as definitively confirming DNA as the genetic material. Students are challenged to contemplate why Avery, MacLeod, and McCarty’s findings did not suffice to convince the scientific community. The teacher underscores the human and social dimensions of science, explaining that disagreement among scientists persisted during Avery, MacLeod, and McCarty’s era. Many clung to the prevailing belief in proteins as the genetic material, attributing errors in methodology to the researchers (Downie ,1972). This insight into scientific disagreement serves as a hallmark to the human and dynamic nature of scientific discourse.
DISCUSSION
Inquiry-Based and HOS Science Teaching coupled with learner engagement in activities relevant to those of scientists constitutes a potent learning environment that fosters the development of both knowledge about the methods and practices through which science progresses and knowledge about desired views of NOS and NOSI (Hrisa & Psillos, 2022; Schwartz et al., 2004).
This study’s approach is consistent with previous literature that emphasizes the importance of contextualizing both scientific concepts and processes within their historical and epistemological frameworks. For example, the study aligns with the findings of Aragón-Méndez et al. (2018) who highlighted the benefits of using historical case studies in science education to enhance students’ understanding of NOS and resonates with Gandolfi’s (2021) view that historical resources can support a more balanced context-based teaching about both epistemic and socio-cultural NOS aspects. Additionally, the integration of NOS and NOSI items into science instruction has been advocated by scholars such as Reiff-Cox (2020), who argued for a more authentic description of SI in educational practices. In this context, the study’s use of Griffith’s experiments as a gateway to explore scientific creativity and unpredictability builds on the insights of Ndeke et al. (2016), who emphasized the critical role of creativity in scientific discovery and the importance of engaging both students and teachers with this often-overlooked aspect of science education.
The instructional proposal outlined in this study can help secondary biology teachers not only illuminate the procedural aspects of experiments ensuring a strong enactment of SI by students but also internalize the instructional importance of NOSI and NOS (Pérez & Díaz-Moreno, 2022). More importantly, the kind of instruction we propose can also help secondary biology teachers portray science as a social and creative process carried out by creative people. Through the use of historical case studies, such as the Griffith experiment, students are exposed to the human elements of SI, including the challenges, uncertainties, and social influences that shape scientific progress. The use of simulated experiments further supports this instructional approach by offering students a hands-on, interactive experience that reinforces the idea of science as a process.
The notion that science is not an objective, non-creative, timeless, and non-social endeavor solely focused on accumulating research facts–thereby implying that scientific knowledge consists of constructed ideas rather than an absolute truth about the world–is a recurring theme in much of the literature on NOS (e.g., Dudu, 2014; Osborne et al., 2003; Patan & Kucuk, 2022). This message is crucial because one of the most widespread misconceptions about NOS, particularly among teachers and students–including Greek physics and biology teachers–is the belief in the myth of the scientific method (Reiff-Cox, 2020; Schizas & Psillos, 2019; Schizas et al., 2023). But why do teachers and students hold this belief, and what are the implications for the concept of “citizenship”?
According to McComas (1996) and other science educators, the belief in the myth of the scientific method arises from the way scientific research is propagated and presented in journals and textbooks. This can be seen as true but only partially. The authors (Schizas & Psillos 2019; Schizas et al., 2020, 2023) demonstrate that Greek physics and biology teachers attribute essential and universal properties to the scientific method because they lack alternative ways to distinguish between the natural/empirical world and the scientific one, and consequently, between science and other social or everyday practices. These teachers are prone to empiricism and naïve realism and face significant challenges in understanding that natural sciences transform the natural world into discipline-specific scientific objects. As a result, they miss a lot of qualities behind these transformations, which could support a realistic and ‘becoming’ definition of what science truly is. In other words, Greek physics and biology teachers fail to recognize that scientific practice is an active, concrete, and socially embedded endeavor, full of multiple and diverse determinations and become vulnerable to viewing the scientific method as an absolute, static, and non-creative process that simply accumulates empirical facts.
From an epistemological perspective, such views elevate empirical facts to things whose operative events can be considered independent of human action. This creates a reified conception of science–as if it were a “thing” that is complete, timeless, and unaffected by historical and social contexts. These views certainly carry social implications. Science loses the traces of its own human production and becomes mystified, scientific know ledge is stripped of any subjective dimension and takes on the role of an authoritative and powerful social force, and, in the end, people become subordinated to the perceived power of “things” and lose sight of the fact that reality is, to some extent, socially constructed.
An open research question persists regarding how science instructions can effectively assist teachers and students in grappling with these outcomes. This study initiates the exploration of this question, proposing an instructional approach that resists presenting SI as a finite and asocial educational outcome. Nevertheless, further research is imperative to theoretically delve into this question and empirically evaluate the extent to which our instruction succeeds in demystifying and de-reifying science.
CONCLUSION
In conclusion, the instructional approach proposed in this study offers a comprehensive framework for teaching science that integrates NOS and NOSI items within an inquiry-based and historically contextualized environment. This method not only addresses the reification of science by presenting it as a dynamic and socially embedded process but also offers a pedagogically robust framework for enhancing students’ understanding of both scientific content and the processes that underpin scientific discovery.
While the study does not include empirical data, the proposed methodology holds significant promise for advancing science education by promoting critical thinking and scientific literacy. By helping students appreciate the complexities and uncertainties inherent in SI, this approach aims to cultivate a deeper and more informed understanding of science, preparing students to engage thoughtfully with scientific issues both within and beyond the classroom.
Author contributions: All authors have sufficiently contributed to the study and agreed with the results and conclusions.
Funding: No funding source is reported for this study.
Ethical statement: The authors stated that the study did not require a formal ethics committee approval letter. No primary data was collected for this study.
Declaration of interest: No conflict of interest is declared by authors.
Data sharing statement: Data supporting the findings and conclusions are available upon request from the corresponding author.
References
- Abd-El-Khalick, F. (2012). Nature of science in science education: Toward a coherent framework for synergistic research and development. In B. J. Fraser, K. Tobin, & C. McRobbie (Eds.), Second international handbook of science education (pp. 1041-1060). Springer. https://doi.org/10.1007/978-1-4020-9041-7_69
- Akerson, V. L., & Donnelly, L. A. (2010). Teaching nature of science to K-2 students: What understandings can they attain? International Journal of Science Education, 32(1), 97-124. https://doi.org/10.1080/09500690902717283
- Akpan, J. P. (2001). Issues associated with inserting computer simulations into biology instruction: A review of the literature. Electronic Journal of Science Education, 5(3).
- Aleporou-Marinou, V., Argirokastritis, A., Komitopoulou, A., Pialoglou, P., & Sgouritsa, V. (1999). Biology. Ministry of Education and Religion.
- Allchin, D. (2011). Evaluating knowledge of the nature of (whole) science. Science Education, 95(3), 918-942. https://doi.org/10.1002/sce.20432
- Aragón-Méndez, M. M., Acevedo-Díaz, J. A., & García-Carmona, A. (2019). Prospective biology teachers’ understanding of the nature of science through an analysis of the historical case of semmelweis and childbed fever. Cultural Studies of Science Education, 14(3), 525-555. https://doi.org/10.1007/s11422-018-9868-y
- Bell, R. L., Mulvey, B. K., & Maeng, J. L. (2012). Beyond understanding: Process skills as a context for nature of science instruction. In M. S. Khine (Ed.), Advances in nature of science research: Concepts and methodologies (pp. 225-245). Springer. https://doi.org/10.1007/978-94-007-2457-0_11
- BSCS. (2009). The biology teacher’s handbook. NSTA Press.
- Capps, D., & Crawford, B. (2013). Inquiry-based instruction and teaching about nature of science: Are they happening? Journal of Science Teacher Education, 24(3), 497-526. https://doi.org/10.1007/s10972-012-9314-z
- Clough, M. P. (2018). Teaching and learning about the nature of science. Science & Education, 27(1-2), 1-5. https://doi.org/10.1007/s11191-018-9964-0
- Dagher, Z. R., & Erduran, S. (2016). Reconceptualizing the nature of science for science education. Why does it matter? Science & Education, 25(1-2), 147-164. https://doi.org/10.1007/s11191-015-9800-8
- Downie, A. W. (1972). Pneumococcal transformation–A backward view fourth Griffith memorial lecture. Microbiology, 73(1), 1-11. https://doi.org/10.1099/00221287-73-1-1
- Dudu, W. T. (2014). Exploring South African high school teachers’ conceptions of the nature of scientific inquiry: A case study. South African Journal of Education, 34(1), Article 782. https://doi.org/10.15700/201412120937
- Gandolfi, H. E. (2021). “It’s a lot of people in different places working on many ideas”: Possibilities from global history of science to Learning about nature of science. Journal of Research in Science Teaching, 58(4), 551-588. https://doi.org/10.1002/tea.21671
- García-Carmona, A. (2021). Improving pre-service elementary teachers’ understanding of the nature of science through an analysis of the historical case of Rosalind Franklin and the structure of DNA. Research in Science Education, 51(2), 347-373. https://doi.org/10.1007/s11165-018-9798-4
- García-Carmona, A., & Acevedo-Díaz, J. A. (2017). Understanding the nature of science through a critical and reflective analysis of the controversy between Pasteur and Liebig on fermentation. Science & Education, 26, 65-91. https://doi.org/10.1007/s11191-017-9876-4
- Gess-Newsome, J. (2002). The use and impact of explicit instruction about the nature of science and science inquiry in an elementary science methods course. Science & Education, 11(1), 55-67. https://doi.org/10.1023/A:1013054823482
- Griffith, F. (1928). The significance of pneumococcal types. Journal of Hygiene, 27(2), 113-159. https://doi.org/10.1017/S0022172400031879
- Hoefnagels, M. (2015). Biology: Concepts and investigations. New York: cGraw-Hill.
- Hrisa, K., & Psillos, D. (2022). Investigating the effectiveness of explicit and implicit inquiry-oriented instruction on primary students’ views about the non-linear nature of inquiry. International Journal of Science Education, 44(4), 604-626. https://doi.org/10.1080/09500693.2022.2050486
- Kampourakis, K. (2016). The ‘general aspects’ conceptualization as a pragmatic and effective means to introducing students to nature of science. Journal of Research in Science Teaching, 53(5), 667-682. https://doi.org/10.1002/tea.21305
- Keeley, P., Eberle, F., & Farrin, L. (2008). Uncovering student ideas in science, volume 3: Another 25 formative assessment probes. National Science Teachers Association.
- Khishfe, R., & Lederman, N. (2007). Relationship between instructional context and views of nature of science. International Journal of Science Education, 29(8), 939-961. https://doi.org/10.1080/09500690601110947
- Kind, P. M., & Kind, V. (2007). Creativity in science education: Perspectives and challenges for developing school science. Studies in Science Education, 43, 1-37. https://doi.org/10.1080/03057260708560225
- Leblebicioglu, G., Capkinoglu, E. Y., Peten, D. M., & Schwartz, R. S. (2020). Views of nature of scientific inquiry of students in different high schools. TED Eğitim ve Bilim, 45(201),143-165. https://doi.org/10.15390/EB.2020.7911
- Leblebicioğlu, G., Metin, D., Çapkınoğlu, E., Çetin, P. S., Eroğlu Doğan, E., & Schwartz, R. (2017). Changes in students’ views about nature of scientific inquiry at a science camp. Science & Education, 26(7-9), 889-917. https://doi.org/10.1007/s11191-017-9941-z
- Lederman, J. S., Lederman, N. G., Bartels, S., Jimenez, J., Acosta, K., Akubo, M., Aly, S., de Andrade, M. B. S., Atanasova, M., Blanquet, E., Blonder, R., Brown, P., Cardoso, R., Castillo-Urueta, P., Chaipidech, P., Concannon, J., Dogan, O. K., El-Deghaidy, H., Elzorkani, A., … Wishart, J. (2021). International collaborative follow-up investigation of graduating high school students’ understandings of the nature of scientific inquiry: Is progress being made? International Journal of Science Education, 43(7), 991-1016. https://doi.org/10.1080/09500693.2021.1894500
- Lederman, J. S., Lederman, N. G., Bartos, S. A., Bartels, S. L., Meyer, A. A., & Schwartz, R. S. (2014). Meaningful assessment of learners’ understandings about scientific inquiry–The views about scientific inquiry (VASI) questionnaire. Journal of Research in Science Teaching, 51(1), 65-83. https://doi.org/10.1002/tea.21125
- Lederman, J., Lederman, N., Bartels, S., Jimenez, J., Akubo, M., Aly, S., Bao, C., Blanquet, E., Blonder, R., de Andrade, M. B. S., Buntting, C., Cakir, M., El-Deghaidy, H., ElZorkani, A., Gaigher, E., Guo, S., Hkanen, A., Al-Lal, S. H., Han-Tosunoglu, C., …, & Zhou, Q. (2019). An international collaborative investigation of beginning seventh-grade students’ understandings of scientific inquiry: Establishing a baseline. Journal of Research in Science Teaching, 56(4), 486-515. https://doi.org/10.1002/tea.21512
- Lederman, N. G. (2018). Nature of scientific knowledge and scientific inquiry in biology teaching. In K. Kampourakis, & M. J. Reiss (Eds.), Teaching biology in schools: Global research, issues, and trends (pp. 216-235). Routledge. https://doi.org/10.4324/9781315110158-18
- Lederman, N. G., & Abd-El-Khalick, F. (1998). Avoiding de-natured science: Activities that promote understandings of the nature of science. In W. F. McComas (Ed.), The nature of science in science education: Rationales and strategies (pp. 83-126). Kluwer Academic. https://doi.org/10.1007/0-306-47215-5_5
- Lederman, N. G., Abd-El-Khalick, F., Bell, R. L., & Schwartz, R. S. (2002). Views of nature of science questionnaire: Toward valid and meaningful assessment of learners’ conceptions of nature of science. Journal of Research in Science Teaching, 39(6), 497-521. https://doi.org/10.1002/tea.10034
- Lederman, N. G., Lederman, J. S., & Antink, A. (2013). Nature of science and scientific inquiry as contexts for the learning of science and achievement of scientific literacy. International Journal of Education in Mathematics, Science and Technology, 1(3), 138-147.
- Lee, A. T., Hairston, R. V., Thames, R., Lawrence, T., & Herron, S. S. (2002). Using a computer simulation to teach science process skills to college biology and elementary majors. Bioscene, 28(4), 35-42.
- Mader, S. (2009). Concepts of biology. McGraw-Hill.
- Mader, S., & Windelspecht, M. (2019). Biology. McGraw-Hill.
- McComas, W. F. (1996). Ten myths of science: Reexamining what we think we know about the nature of science. School Science and Mathematics, 96(1), 10-16. https://doi.org/10.1111/j.1949-8594.1996.tb10205.x
- Mesci, G. (2016). Preservice science teachers’ pedagogical content knowledge for nature of science and nature of scientific inquiry: A successful case study [Doctoral dissertation, Western Michigan University].
- Mesci, G., & Schwartz, R. (2017). Changing preservice science teachers’ views of nature of science: Why some conceptions may be more easily altered than others. Research in Science Education, 47(2), 329-351. https://doi.org/10.1007/s11165-015-9503-9
- Mesci, G., Çavuş-Güngören, S., & Yesildag-Hasancebi, F. (2020). Investigating the development of pre-service science teachers’ NOSI views and related teaching practices. International Journal of Science Education, 42(1), 50-69. https://doi.org/10.1080/09500693.2019.1700316
- Michel, H., & Neumann, I. (2016). Nature of science and science content learning. Science & Education, 25(9-10), 951-975. https://doi.org/10.1007/s11191-016-9860-4
- Ndeke, G. C., Okere, M. I., & Keraro, F. N. (2016). Secondary school biology teachers’ perceptions of scientific creativity. Journal of Education and Learning, 5(1), 31-43. https://doi.org/10.5539/jel.v5n1p31
- NGSS Lead States. (2013). Next generation science standards: For states, by states. The National Academies Press.
- Osborne, J., Collins, S., Ratcliffe, M., Millar, R., & Duschl, R. (2003). What “ideas-about-science” should be taught in school science? A Delphi study of the expert community. Journal of Research in Science Teaching, 40(7), 692-720. https://doi.org/10.1002/tea.10105
- Patan, A., & Kucuk, M. (2022). The influence of imagination and creativity-based science teaching on Turkish middle school students’ nature of science views. Education Quarterly Reviews, 5(2), 707-719. https://doi.org/10.31014/aior.1993.05.04.654
- Penn, M., Ramnarain, U., Kazeni, M., Dhurumraj, T., Mavuru, L., & Ramaila, S. (2021). South African primary school learners’ understandings about the nature of scientific inquiry. Education 3-13 International Journal of Primary, Elementary and Early Years Education, 49(3), 263-274. https://doi.org/10.1080/03004279.2020.1854956
- Pérez, B. C., & Díaz-Moreno, N. (2022). Promoting pre-service primary teachers’ development of NOSI through specific immersion and reflection. Eurasia Journal of Mathematics, Science and Technology Education, 18(3), Article em2089. https://doi.org/10.29333/ejmste/11795
- Psillos, D. (2023). The role and impact of virtual laboratories in physics teaching and learning: A synthesis of literature. In M. F. Tasar & P. R. L. Heron (Eds.), The International Handbook of Physics Education Research. AIP Publishing. https://doi.org/10.1063/9780735425712_002
- Raven P., Johnson G., Mason K., Losos J., & Singer S. (2017). Biology. McGraw Hill.
- Reiff-Cox, R. (2020). Exchanging the myth of a step-by-step scientific method for a more authentic description of inquiry in practice. In W. F. McComas (Ed.), Nature of science in science instruction (pp. 127-139). Springer. https://doi.org/10.1007/978-3-030-57239-6_6
- Rutten, N., Van Joolingen, W. R., & Van Der Veen, J. T. (2012). The learning effects of computer simulations in science education. Computers & Education, 58(1), 136-153. https://doi.org/10.1016/j.compedu.2011.07.017
- Sadava, D., Hillis, D., Heller, H., & Hacker, D. (2016). Life: The science of biology. Sinauer Associates.
- Schizas, D., & Psillos, D. (2019). Exploring physics teachers’ NOTSs (Nature Of The Sciences) conceptions and discussing their relation to the current domain-general NOS (Nature Of Science) agenda. The Electronic Journal for Research in Science & Mathematics Education, 23(2), 19-48.
- Schizas, D., Psillos, D., & Papadopoulou, P. (2022). Bonding Nature of Science (NOS) and Nature of the Sciences (NOTSs) with Conceptual Knowledge: Introducing NOS and NOTSs Learning Objectives into the Teaching of ‘Homeostasis’. Interdisciplinary Journal of Environmental and Science Education, 18(4), e2298. https://doi.org/10.21601/ijese/12311
- Schizas, D., Psillos, D., & Stamou, G. (2016). Nature of science or nature of the sciences?. Science Education, 100(4), 706-733. https://doi.org/10.1002/sce.21216
- Schizas, D., Psillos, D., & Stamou, G. (2020). Exploring secondary school biology teachers’ conceptions of explanations. International Journal of Biological Education, 2(1), 22-32.
- Schizas, D., Psillos, D., & Stamou, G. (2023). Exploring secondary school biology teachers’ conceptions of scientific laws and methods. International Journal of Science Education, 45(7), 503-520. https://doi.org/10.1080/09500693.2023.2166373
- Schwartz, R. S., Lederman, N. G., & Crawford, B. A. (2004). Developing views of nature of science in an authentic context: An explicit approach to bridging the gap between nature of science and scientific inquiry. Science Education, 88(4), 610-645. https://doi.org/10.1002/sce.10128
- Schwartz, R. S., Lederman, N. G., & Lederman, J. S. (2008). An instrument to assess views of scientific inquiry: The VOSI questionnaire [Paper presentation]. The National Association for Research in Science Teaching.
- Senler, B. (2015). Middle school students’ views of scientific inquiry: An international comparative study. Science Education International, 26(2), 166-179.
- Solomon E., Berg L., & Martin, D. (2011). Biology. Brooks/Cole.
- Stamou, G. P. (2012). Critical realism and ecological studies. In G. P. Stamou (Ed.), Populations, biocommunities, ecosystems: A review of controversies in ecological thinking (pp. 3-27). Bentham Science. https://doi.org/10.2174/978160805280611201010003
- Thomas, N. J., & Vo, T. (2021). Using simulations to support undergraduate elementary preservice teachers’ biological understanding of natural selection. Bioscene: Journal of College Biology Teaching, 47(1), 29-39.
- Urry, L., Cain, M., Wasserman, S., Minorsky, P., & Reece, J. (2017). Cambell biology. Pearson.
- Vorholzer, A., Von Aufschnaiter, C., & Boone, W. J. (2020). Fostering upper secondary students’ ability to engage in practices of scientific investigation: A comparative analysis of an explicit and an implicit instructional approach. Research in Science Education, 50, 333-359. https://doi.org/10.1007/s11165-018-9691-1
- Wacker, C. C., Barth, M., Stahl, C., & Schlüter, K. (2022). Toward a questionnaire to assess biology student teachers’ knowledge of the nature of scientific inquiry (NOSI). In Current research in biology education: Selected papers from the ERIDOB community (pp. 59-70). Springer. https://doi.org/10.1007/978-3-030-89480-1_5
- Wallace, J. (2017). Teaching NOS in an age of plurality. Canadian Journal of Science, Mathematics and Technology Education, 17(1), 1-2. https://doi.org/10.1080/14926156.2016.1271925
- Yang, I. H., Park, S. W., Shin, J. Y., & Lim, S. M. (2017). Exploring Korean middle school students’ view about scientific inquiry. Eurasia Journal of Mathematics, Science and Technology Education, 13(7), 3935-3958. https://doi.org/10.12973/eurasia.2017.00765a
How to cite this article
APA
Schizas, D., Psillos, D., & Asimopoulos, S. (2024). Advancing scientific literacy: Integrating nature of science and nature of scientific inquiry in teaching Griffith’s and Avery-MacLeod-McCarty experiments. Interdisciplinary Journal of Environmental and Science Education, 20(4), e2421. https://doi.org/10.29333/ijese/15522
Vancouver
Schizas D, Psillos D, Asimopoulos S. Advancing scientific literacy: Integrating nature of science and nature of scientific inquiry in teaching Griffith’s and Avery-MacLeod-McCarty experiments. INTERDISCIP J ENV SCI ED. 2024;20(4):e2421. https://doi.org/10.29333/ijese/15522
AMA
Schizas D, Psillos D, Asimopoulos S. Advancing scientific literacy: Integrating nature of science and nature of scientific inquiry in teaching Griffith’s and Avery-MacLeod-McCarty experiments. INTERDISCIP J ENV SCI ED. 2024;20(4), e2421. https://doi.org/10.29333/ijese/15522
Chicago
Schizas, Dimitrios, Dimitris Psillos, and Stefanos Asimopoulos. "Advancing scientific literacy: Integrating nature of science and nature of scientific inquiry in teaching Griffith’s and Avery-MacLeod-McCarty experiments". Interdisciplinary Journal of Environmental and Science Education 2024 20 no. 4 (2024): e2421. https://doi.org/10.29333/ijese/15522
Harvard
Schizas, D., Psillos, D., and Asimopoulos, S. (2024). Advancing scientific literacy: Integrating nature of science and nature of scientific inquiry in teaching Griffith’s and Avery-MacLeod-McCarty experiments. Interdisciplinary Journal of Environmental and Science Education, 20(4), e2421. https://doi.org/10.29333/ijese/15522
MLA
Schizas, Dimitrios et al. "Advancing scientific literacy: Integrating nature of science and nature of scientific inquiry in teaching Griffith’s and Avery-MacLeod-McCarty experiments". Interdisciplinary Journal of Environmental and Science Education, vol. 20, no. 4, 2024, e2421. https://doi.org/10.29333/ijese/15522